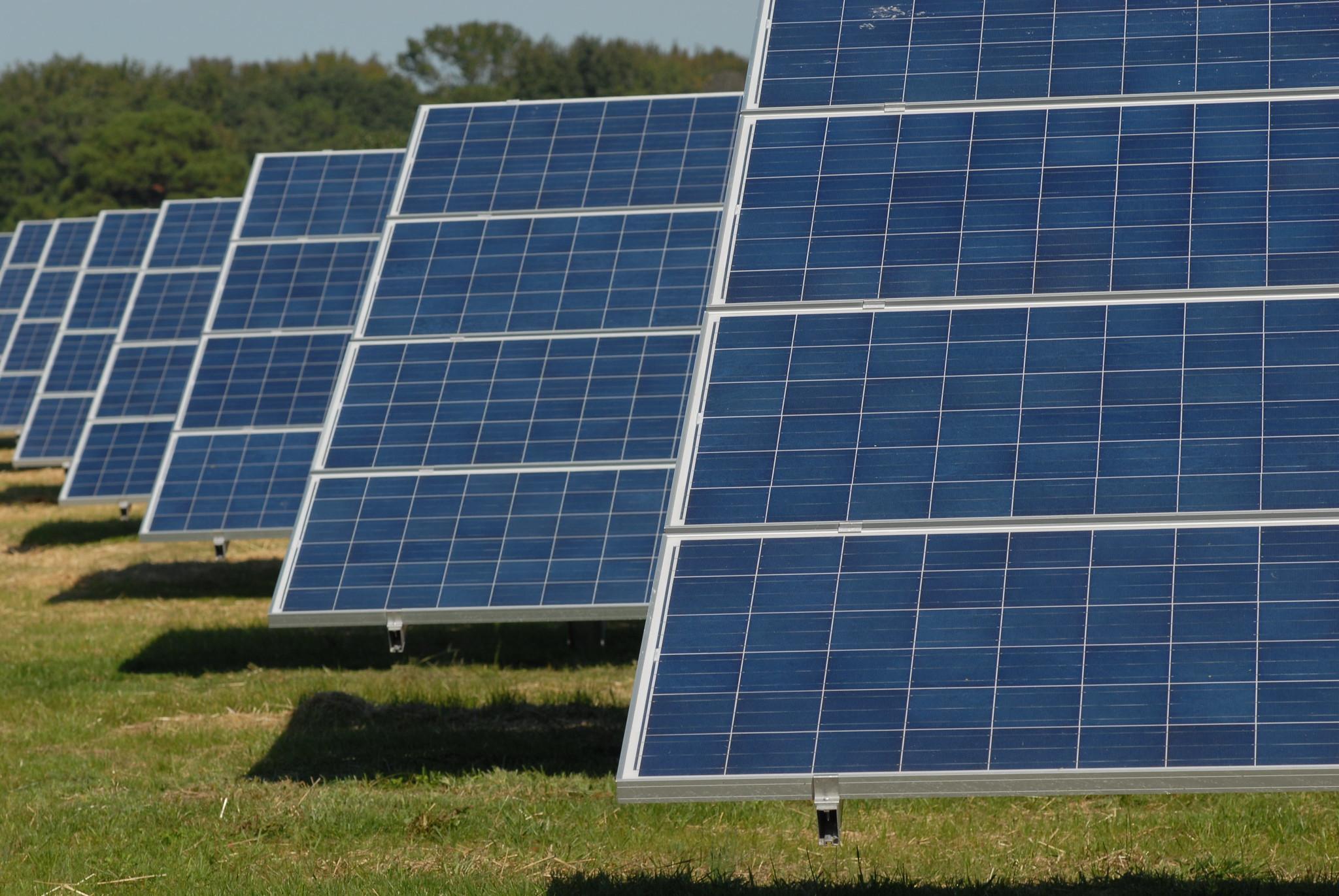
In 2019, the top four energy sources consumed in Maryland were petroleum (35.0%), natural gas (23.0%), nuclear electric power (11.6%), and coal (5.7%). The supply of these non-renewable energy sources is limited by how much we can mine or extract from the earth. An alternative is to use the sun’s energy as the fuel to produce electricity. This is accomplished using photovoltaic (PV) technology. Solar PV is considered a mature technology with large investments and broad adoption across the United States and in Maryland. Between 2010 and 2020, installed solar capacity increased over 100 times for Maryland alone. As of 2021, solar energy accounts for over 6% of U.S. renewable electricity and over 38% of Maryland’s renewable electricity. Roughly two-thirds of Maryland’s solar generation is derived from small-scale solar PV (e.g., rooftop systems), with utility-scale projects (i.e., solar farms) accounting for the remainder. By early 2021, Maryland had installed over 1,200 megawatts (MW) of solar-generating capacity.
The letters PV stand for “Photo” = light and “Voltaics” = electricity. PV technology can potentially be used anywhere the sun shines. Solar electricity is produced through the PV Effect: when sunlight hits a solar cell, electrons are released and flow as electricity through wires to your building or equipment. Solar cells are connected together to form a panel (also called a module). Panels are wired together to form an array. PV technology is used to power everything from calculators and outdoor lighting fixtures to buildings and satellites. Whatever is powered by electricity (appliances, machinery, etc.) is called the electrical “load.” The sun can provide electricity for your home, greenhouse, and barn. It can also electrify fences and pump water. In addition to the PV panels, a solar electric system (also called a PV system) includes an inverter, meter, and safety equipment. It may include batteries and a charge controller. These systems contain no moving parts, are silent, very durable and reliable, and are low maintenance. Once installed, they only use the sun’s energy and their operation produces no emissions. Solar electric systems can produce all or a portion of the electricity needed. PV panels can be added to an existing system over time.